The “Chemical Theory Group” of ALGC is developing and implementing chemical concepts and theories for challenging applications, involving the investigation of chemical reactivity, molecular properties and the rational design of molecular compounds using quantum chemical calculations and advanced computer simulations. Applications are almost always conducted in direct collaboration with experimental groups. The group’s activities are centered around 8 major research lines highly connected and mutually reinforcing research tracks: Conceptual DFT, Single-Molecule Electronics, Molecular Switches, Chemical Reactivity in Solution, Inverse Molecular Design, Aromaticity, Multiscale Modeling, and Main-Group Metal Catalysis.
Conceptual DFT
Within the context of Density Functional Theory (DFT), many very popular but previously often vaguely defined chemical concepts, such as electronegativity, hardness and softness, useful in describing and interpreting atomic, molecular and material electronic structure and reactivity were sharply defined. This branch of DFT was termed “Conceptual DFT” or “Density Functional Reactivity Theory” and has been the central theme of research of the ALGC group for more than 30 years. “Conceptual DFT” is based on the fact that when a molecule is involved in a chemical reaction, it ultimately undergoes a change in its number of electrons N and/or its external potential ν(r). The energy variation upon these changes can be quantified by a Taylor series expansion in which the energy derivatives are associated to chemical concepts, thus naturally providing a connection between energy changes and concepts. In this part of our research, we develop, implement, and use chemical reactivity concepts and principles and apply them to a variety of different chemical systems with ranging complexity. In addition, we also use other interpretational tools from quantum chemistry in these studies.
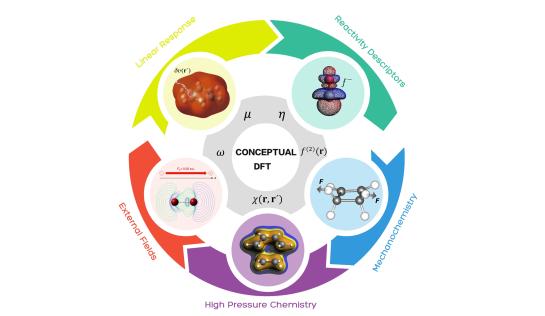
Highlighted publications
P. Geerlings, F. De Proft, W. Langenaeker, Conceptual density functional theory, Chem. Rev. 2003, 103, 1793–1874. doi:10.1021/cr990029p
P. Geerlings, S. Fias, Z. Boisdenghien, F. De Proft, Conceptual DFT: Chemistry from the linear response function, Chem. Soc. Rev. 2014, 43, 4989–5008. doi:10.1039/c3cs60456j
T. Clarys, T. Stuyver, F. De Proft, P. Geerlings, Extending conceptual DFT to include additional variables: Oriented external electric field, Phys. Chem. Chem. Phys. 2021, 23, 990–1005. doi:10.1039/D0CP05277A
Single-Molecule Electronics
Creating functional nanoscale devices using single molecules as active electronic components is the ultimate goal of the field of molecular electronics. In recent years, we focus on understanding the different factors that control electron transport through single molecules and the thermoelectric properties of molecular junctions. We are interested in quantum interference effects and how simple changes in the structure of the electronically active molecule and the connectivity to metallic electrodes can led to dramatically different transport properties. By starting from the source-and-sink potential model at the Hückel level of theory, we devise different selection rules to successfully predict the presence of quantum interferences in Hückel and Möbius polyenes. Different chemical concepts are exploited in the design of novel single-electronic devices, including aromaticity, diradical character, molecular topology and different π-conjugation modes. By linking chemical concepts and quantum chemical descriptors with molecular conductance, a chemical understanding of such complex physical phenomena becomes possible.
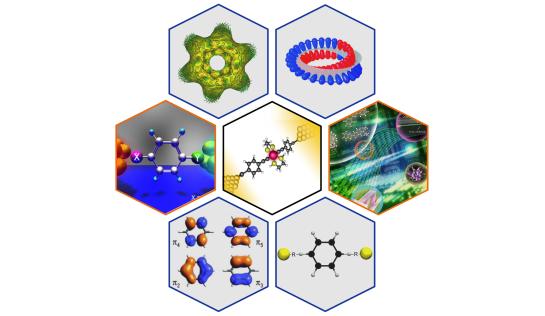
Highlighted publications
J. Gu, W. Wu., T. Stuyver, D. Danovisch, R. Hoffmann, Y. Tsuji, S. Shaik, Cross conjugation in polyenes and related hydrocarbons: What can be learned from valence bond theory about single-molecule conductance?, J. Am. Chem. Soc. 2019, 141, 6030–6047. doi:10.1021/jacs.9b01420
T. Stuyver, S. Fias, P. Geerlings, F. De Proft, M. Alonso, Qualitative insights into the transport properties of Hückel/Möbius (anti)aromatic compounds: Application to expanded porphyrins, J. Phys. Chem. C 2018, 122, 19842–19856. doi:10.1021/acs.jpcc.8b01424
T. Stuyver, T. Zeng, Y. Tsuji, P. Geerlings, F. De Proft, Diradical character as a guiding principle for the insightful design of molecular nanowires with an increasing conductance with length, Nano Lett. 2018, 18, 7298–7304. doi:10.1021/acs.nanolett.8b03503
Molecular Switches
The design of molecular switches based on p-conjugated systems constitutes an important research topic. We have implemented a bottom-up quantum chemical approach to investigate the numerous factors controlling the switching behavior, not only at the single-molecule level but also in the device architecture. A novel type of molecular switches based on redox and topology interconversions in expanded porphyrins was recently discovered for different nanoelectronic applications, including conductance switching, bithermoelectric devices and nonlinear optical switches. We are interested in the mechanical activation of molecular switches and the application of inverse design techniques to explore the combinatorial chemical compound space of expanded porphyrins and photoswitches in search of high contrast optical switches.
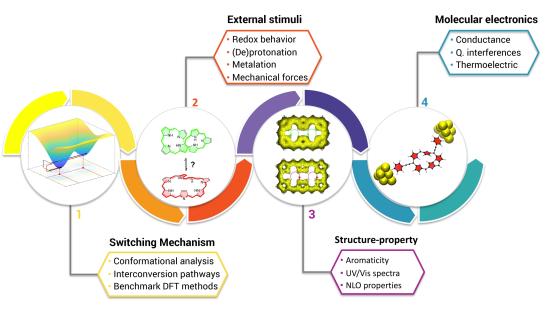
Highlighted publications
T. Stuyver, M. Perrin, P. Geerlings, F. De Proft, M. Alonso, Conductance switching in expanded porphyrins through aromaticity and topology changes, J. Am. Chem. Soc. 2018, 140, 1313–1326. doi:10.1021/jacs.7b09464
T. Stuyver, P. Geerlings, F. De Proft, M. Alonso, Toward the design of bithermoelectric switches, J. Phys. Chem. C 2018, 122, 24436–24444. doi:10.1021/acs.jpcc.8b07753
T. Bettens, M. Hoffmann, M. Alonso, P. Geerlings, A. Dreuw, F. De Proft, Mechanochemically triggered topology changes in expanded porphyrins, Chem. Eur. J. 2021, 27, 3397–3406. doi:10.1002/chem.202003869
Chemical Reactivity in Solution
In recent years, we have used advanced ab initio molecular dynamics methods, such as metadynamics, to explore chemical reactivity in solution. For instance, we have focused on electrophilic aromatic substitution, where we further scrutinized the rich mechanistic landscape of this reaction for chlorination and sulfonation. Recently, in a collaboration with the organic chemistry research group of the VUB (ORGC), a combined theoretical/experimental study was conducted on the halolactonization reaction, a common type of electrophilic addition to alkenes. Using ab initio metadynamics simulations, the halolactonization reaction of alkenoic acids was investigated and two different pathways, the classical textbook two-step mechanism and the recently proposed concerted nucleophile-assisted alkene addition mechanism, were scrutinized in different solvents.
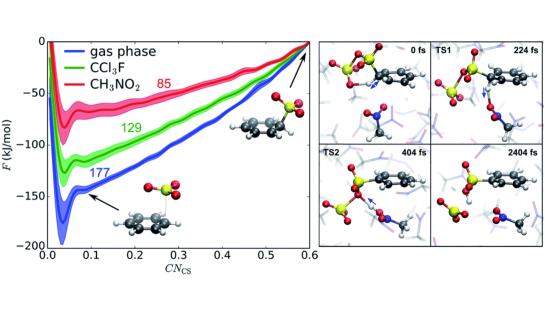
Highlighted publications
S.L.C. Moors, X. Deraet, G. Van Assche, P. Geerlings, F. De Proft, Aromatic sulfonation with sulfur trioxide: Mechanism and kinetic model, Chem. Sci. 2017, 8, 680–688. doi:10.1039/C6SC03500K
R. Van Lommel, S.L.C. Moors, F. De Proft, Solvent and autocatalytic effects on the stabilisation of the σ-complex during electrophilic aromatic chlorination, Chem. Eur. J. 2018, 27, 7044–7050. doi:10.1002/chem.201800385
R. Van Lommel, J. Bock, D.G. Constantin, U. Hennecke, F. De Proft, A dynamic picture of the halolactonization reaction through a combination of ab initio metadynamics and experimental investigations, Chem. Sci. 2021, 12, 7746–7757. doi:10.1039/D1SC01014
Inverse Molecular Design
The rapid increase in computing power and speed not only impacts the accuracy of quantum-chemical calculations, but also opens doors to a more exhaustive exploration of chemical compound space by means of artificial intelligence approaches and inverse design techniques. Molecules or materials with tailored properties can be inversely designed by considering the desired functionality or reactivity as the starting point of the design process. Chemical modifications to the molecular structure or material are accepted if they lead to an improvement of the target property. Machine-learning techniques can be integrated in the process to accelerate the CCS search by property prediction. In recent years, an in-house inverse design program package was developed to perform property optimizations using both combinatorial and stochastic approaches. Current applications focus on molecular switches, redox-flow batteries, organic materials in (opto)electronics, acid-base chemistry, and radical reactivity.
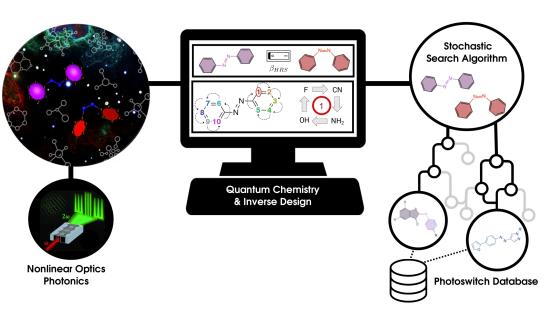
Highlighted publications
E. Desmedt, D. Smets, T. Woller, M. Alonso, F. De Vleeschouwer, Designing hexaphyrins for high-potential NLO switches: The synergy of core-modifications and meso-substitutions, Phys. Chem. Chem. Phys. 2023, 25, 17128–17142. doi:10.1039/D3CP01240A
J.L. Teunissen, F. De Proft, F. De Vleeschouwer, Tuning the HOMO-LUMO gap of small diamondoids using inverse molecular design, J. Chem. Theory Comput. 2017, 13, 1351–1365. doi:10.1021/acs.jctc.6b01074
F. De Vleeschouwer, A. Chankisjijev, W. Yang, P. Geerlings, F. De Proft, Pushing the boundaries of intrinsically stable radicals: inverse design using the thiadiazinyl radical as a template, J. Org. Chem. 2013, 78, 3151-3158. doi:10.1021/jo400101d
Aromaticity
In the field of aromaticity, we have introduced a multidimensional approach to quantify Hückel and Möbius aromaticity on macrocycles, based on global and local descriptors rooted on the energetic, magnetic, reactivity, structural and electronic criteria. Neural networks and advanced multidimensional scaling techniques are applied for the clustering, visualization, and quantification of aromaticity of a large database of chemical systems considering the different manifestations of the concept of aromaticity. By using these novel descriptors, we have established the complex structure-property relationships between aromaticity, π-conjugation topology and photophysical properties. Through this research, we have shown that the concept of aromaticity has a profound impact on the rationalization of molecular properties and reactivity of countless systems and is a highly valuable guiding principle in the design of new fascinating molecules and materials, such as single-molecule optoelectronic devices.
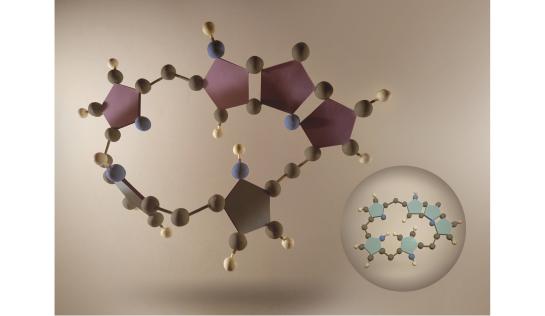
Highlighted publications
M. Alonso, I. Fernández, in Aromaticity: Modern computational methods and applications, ed. I. Fernández, Elsevier, Amsterdam, 1st edn, 2021, ch. 6, pp. 195–235. ISBN:9780128227237
T. Woller, P. Geerlings, F. De Proft, B. Champagne, M. Alonso, The fingerprint of aromaticity and molecular topology on the photophysical properties of octaphyrins, J. Phys. Chem. C 2019, 123, 7318–7335. doi:10.1021/acs.jpcc.8b10908
I. Casademont-Reig, T. Woller, J. Contreras-García, M. Alonso, M. Torrent-Sucarrat, E. Matito, New electron delocalization tools to describe the aromaticity in porphyrinoids, Phys. Chem. Chem. Phys. 2018, 20, 2787–2796. doi:10.1039/C7CP07581B
Multiscale Modeling
In recent years, we are developing multiscale modeling approaches integrating quantum chemistry, molecular dynamics and conceptual approaches to understand and even predict complex chemical processes. A recent example involves supramolecular gelation in which the integration of different computational tools with different levels of accuracy is required to gain information at different time and length scales. By integrating QM methods, MD simulations and machine learning techniques, we developed a model to predict the gelation propensity of a molecule in both organic solvents and water. Currently, we are developing reactive MD simulation models for the self-healing after damage in covalent adaptable networks through reversible Diels-Alder reactions. We aim to suggest improved self-healing materials that can tremendously extend the lifetime and durability of a product. Other applications involved the simulations of deep eutectic solvents, solid state battery materials and the prediction of polymer solubility.
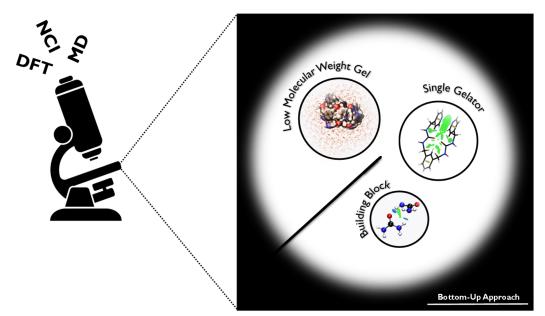
Highlighted publications
R. Van Lommel, J. Zhao, W.M. De Borggraeve, F. De Proft, M. Alonso, Molecular dynamics based descriptors for predicting supramolecular gelation, Chem. Sci. 2020, 11, 4226–4238. doi:10.1039/D0SC00129E
M.H. Mamme, S.L. Moors, H. Terryn, J. Deconinck, J. Ustarroz, F. De Proft, Atomistic insight into the electrochemical double layer of choline chloride–urea deep eutectic solvents: Clustered interfacial structuring, J. Phys. Chem. Lett. 2018, 9, 6296–6304. doi:10.1021/acs.jpclett.8b01718
M. Denayer, J. Vekeman, F. Tielens, F. De Proft, Towards a predictive model for polymer solubility using the non-covalent interaction index: Polyethylene as a case study, Phys. Chem. Chem. Phys. 2021, 23, 25374–25387. doi:10.1039/D1CP04346C
Main-Group Metal Catalysis
The field of main-group metal catalysis is of great current interest due to its potential to circumvent the costly and, often harmful, transition metal catalysts. We are interested in the characterization of the electronic structure and bonding of reactive main group species as well as the reaction mechanism by means of computational methods. Our aim is to establish guiding principles for the design of main-group metals for homogeneous catalysis. The predictions are usually benchmarked by proof-of-principle experiments. The most recent examples are the development of efficient simple alkaline-earth-metal catalysts for effective imine and alkene hydrogenations and the search for novel catalysts for the ring-opening polymerizations of cyclic esters based on cationic group 14 metal complexes.
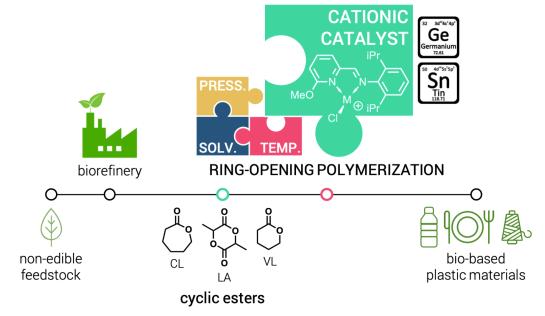
Highlighted publications
N-Donor stabilized tin(II) cations as efficient ROP catalysts for the synthesis of linear and star-shaped PLAs via the activated monomer mechanism, Dalton Trans. 2021, 50, 16039–16052. doi:10.1039/D1DT02658E
H. Bauer, M. Alonso, C. Färber, H. Elsen, J. Pahl, A. Causero, G. Ballmann, F. De Proft, S. Harder, Imine hydrogenation with simple alkaline-earth metal catalysts, Nat. Catal. 2018, 1, 40–47. doi:10.1038/s41929-017-0006-0
H. Bauer, M. Alonso, C. Fischer, B. Rösh, H. Elsen, S. Harder, Simple alkaline-earth metal catalysts for effective alkene hydrogenation, Angew. Chem. Int.Ed. 2018, 57, 15177–15182. doi:10.1002/anie.201810026